Stemming from a relatively recent explosion of research, the epigenetic regulation of gene expression is now appreciated as an important biological phenomenon. The prefix “epi-” means above or around in Greek, as epigenetics is defined by the study of DNA modifications that do not change the underlying sequence of the DNA. Importantly, these additions change cellular gene expression to diversify the functionality of cells with the same underlying genetic code.
Chemical modifications to DNA such as methyl groups (mCpG) are dynamically regulated to govern cell fate during development. The family of DNA methyltransferase enzymes, or DNMTs, covalently add mCpGs to DNA to allow cells to change their transcriptional output. These epigenetic marks can also be erased through either the downregulation of DNMTs and subsequent DNA replication, or through the activation of the Ten-Eleven Translocation (TET) family of enzymes to begin multi-step oxidation of mCpGs. During mammalian germ cell formation in early embryonic development, DNA methylation is dynamically regulated during the process of epigenetic reprogramming. First, the DNA methylation patterns of the egg and sperm are erased, leading to the formation of a totipotent zygote. Next, tissue specific DNA methylation occurs to guide differentiation. Later, these marks too are erased when the primordial germ cells (PGCs) migrate from the epiblast and differentiate into cells with a germ fate.
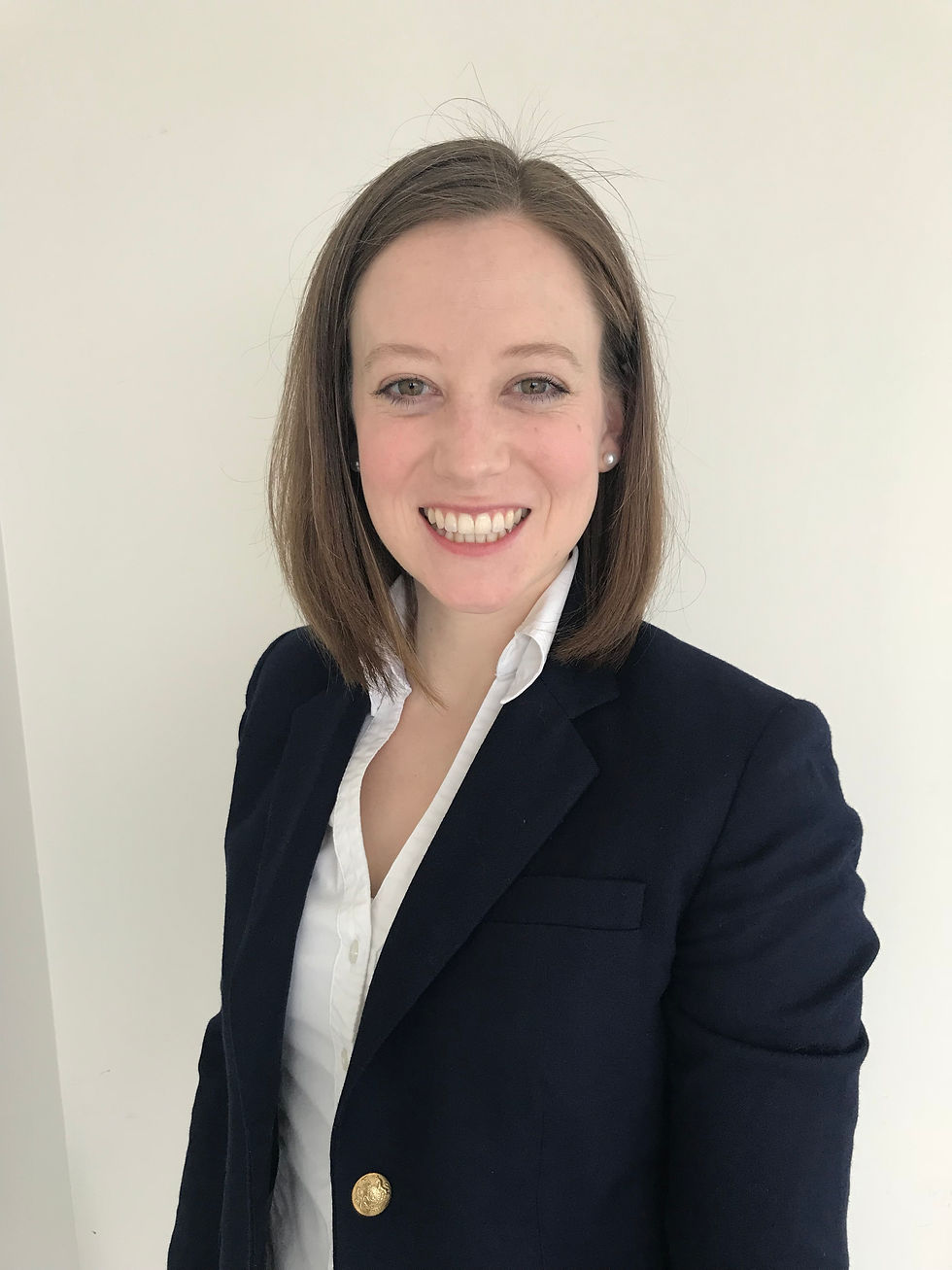
While the establishment of DNA methylation is well-characterized, much less is known about the requirement for DNA demethylation during development. When CAMB G&E alum Jennifer SanMiguel joined the Bartolomei lab, research on the function of TET enzymes in DNA demethylation during development was already underway. Jen was particularly interested in using previously developed Tet1 null mice to examine DNA methylation changes at important developmental loci called imprinting control regions, or ICRs. These regions of the genome are unique because their methylation pattern controls genes that are expressed in a mono-allelic fashion, from either the maternal or the paternal parental copy. One example of this unique regulation is the H19/Igf2 locus, where paternal ICR hypermethylation governs paternal-specific expression of Igf2 and maternal-specific expression of H19.
To understand how TETs function in germ cells, Jen set out to examine how loss of Tet1 affects DNA methylation of ICRs in both male and female germ cells using Tet1 null (KO) offspring, as Tet1 is the most highly expressed TET family member in the germline. Jen hypothesized that TET1 is essential for setting up proper allele-specific patterns of DNA methylation at ICRs. To test this, Jen first examined imprinted methylation with pyrosequencing in female oocytes from pups generated by mating heterozygous Tet1 (het) mutant animals. Interestingly, two loci that are paternally hypermethylated (H19/Igf2 and IG-DMR) had unusually stochastic DNA methylation in the female germline, with up to ~75% DNA methylation in the normally hypomethylated maternal allele. To determine if the abnormal methylation at ICRs in oocytes affects development or gestation, Jen quantified the number of live versus resorbed embryos at E10.5 in matings with either Het or KO Tet1 females to wild type (WT) males. A major hurdle Jen faced during data collection was getting the female Tet1 mice to breed.
She described that, “I spent months breeding heterozygous pairs of mice to get null females, and when I finally got null females, they were almost impossible to successfully mate. I waited almost an entire year between my second litter and my third litter of maternal knockout mice. It was really important for us to have a decent sample size as well as making sure there were no litter specific effects, so waiting for these mice to breed was painful. Luckily, persistence paid off!” Over time, Jen observed that the number of live embryos decreased while the corresponding number of resorbed embryos increased in embryos generated with Tet1 KO female mice, consistent with the idea that the proper demethylation of ICRs is essential for embryonic development.
To determine if abnormal ICR methylation affects imprinted gene expression, Jen used Tet1 KO females bred to WT males harboring multiple single nucleotide polymorphisms at the ICR of imprinted H19/Igf2 for allele-specific analyses. In WT mice at this locus, paternal ICR hypermethylation dictates exclusive Igf2 expression from the paternal allele, while H19 is expressed from the maternal allele. Remarkably, three embryos derived from independent litters of Tet1 KO females had abnormal biallelic expression of Igf2. In agreement, embryos with biallelic expression of Igf2 also had methylation of both the maternal and paternal alleles of the ICR at this locus. Because Igf2 was expressed from both maternal and paternal alleles, Jen observed a sharp decrease in the expression of H19 from both alleles, further suggesting that TET1-mediated germline ICR methylation is required for allele-specific gene expression at the H19/Igf2 locus .
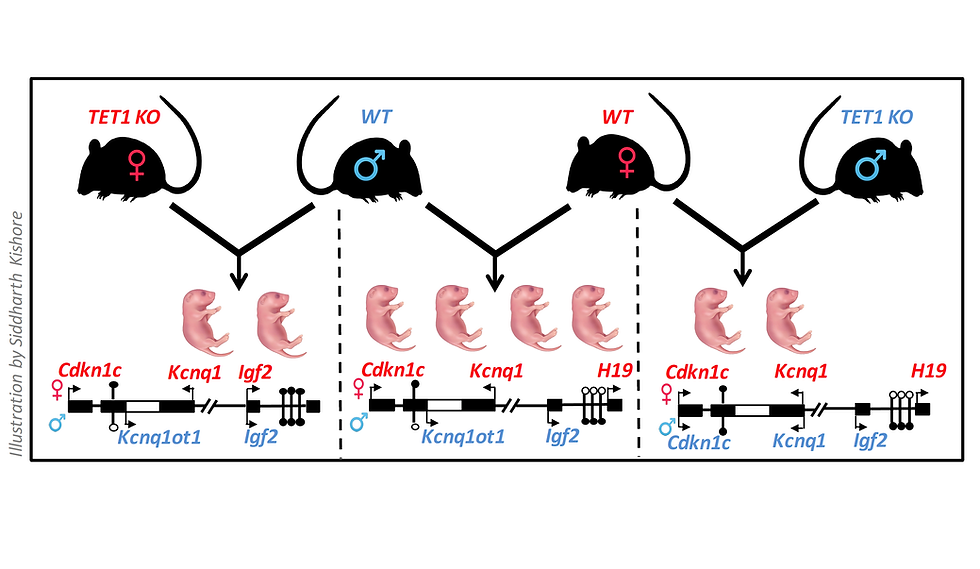
Mating TET1 knockout (KO) males or females their wild type (WT) counter-parts results in fewer live embryos compared to WT controls. This is caused by abnormal DNA methylation at important developmental loci called imprinting control regions (ICR’s). Specifically, when TET1 KO females mate with WT males, the resulting embryos fail to lose DNA methylation marks on the maternally derived allele at the H19/Igf2 locus, which leads to the expression of Igf2 from both the maternal and paternal alleles. Conversely when TET1 KO males mate with WT females, the resulting embryos fail to lose DNA methylation marks at the paternally derived allele at the KvDMR locus, which leads to the expression of Cdkn1c and Kcnq1 from both the maternal and paternal alleles.
Finally, to investigate the effects of Tet1 deletion on the male germline, Jen examined imprinted methylation in sperm from Tet1 Het matings. Similar to observations in Tet1 KO oocytes, Tet1 null sperm had increased methylation of ICRs that are usually maternally hypermethylated and paternally hypomethylated, such as Peg3 and KvDMR. A similar decrease in the number of live births and increase in resorbed embryos was also observed. To determine how the unusual methylation pattern in sperm affects imprinted gene expression of offspring, Jen again analyzed allele-specific expression, this time at the KvDMR ICR. At this locus, Cdkn1c is expressed from the maternal allele in WT animals, but in animals generated with Tet1 KO males, biallelic expression of this gene was observed in multiple adult tissues.
Taken together, Jen and colleagues have demonstrated that Tet1 is essential for establishing proper DNA methylation patterns at critical ICRs in both male and female germ cells. Moreover, without the appropriate imprinted DNA methylation, biallelic expression is observed stochastically in a distinct set of ICRs. Importantly, the loss of imprinted gene expression at ICRs is observed in human imprinting disorders, and this study establishes Tet1 as an important regulator of imprinting in both sexes. As Jen begins her postdoctoral fellowship at the Jackson Laboratory in Bar Harbor, ME, she looks back on her time in CAMB.
“I think my favorite part of my Ph.D. was the community. I was luckily to have a caring and supportive mentor and thesis committee, amazing, caring lab mates, great technical support from the epigenetics community and people on the 9th floor or Smilow and a great connection with my peers and the faculty in G&E. I really loved being surrounded by so many smart, positive, insightful people and it really made my research and my life outside of grad school that much better.”
SanMiguel JM, Abramowitz LK, Bartolomei MS. (2018). Imprinted gene dysregulation in a Tet1 null mouse model is stochastic and variable in the germline and offspring. Development. 2018, 145, dev160622 .