Yee Hoon Foong and Sylvia Stankov
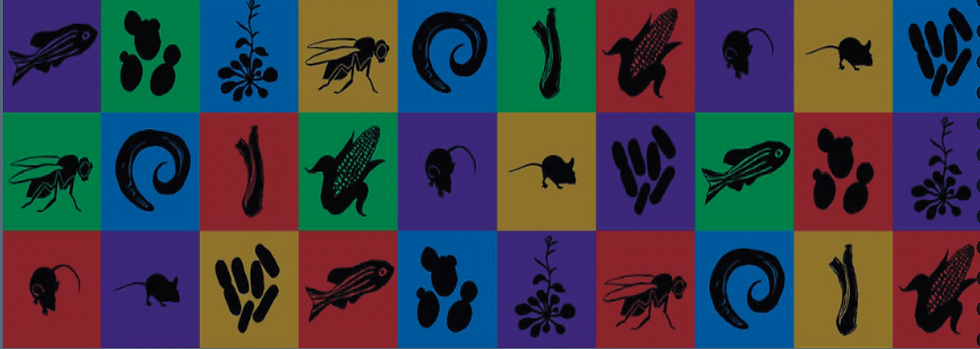
Model systems are an invaluable tool in the biomedical sciences. They serve as controlled stand-ins when asking questions that would otherwise be impossible to explore in humans. Their vast utility is also matched by their vast diversity. Model organisms range from the humble bacterium E. coli to mammals, including mice and non-human primates.
The murine model has traditionally been the preferred model system to study human diseases, while non-murine systems are often underappreciated as less robust counterparts 1,2,3. A meta-analysis of NIH R01 funding trends from 2008 to 2015 showed a drastic disparity in the award rate between murine and non-murine models: each non-murine model organism makes up only 1-2% of total R01 awards, whilst more than 50% of R01 funding is granted to murine models 4,5. Dr. Michael Hart in Penn’s Department of Genetics agrees that “perceptions can create discrepancies in funding between different organisms.”
This article serves to shine a light on the great potential and unprecedented opportunities of non-murine systems in human disease modeling. We spoke with three Penn PIs on their work using non-murine model organisms. Read below for their full interviews!
What are your research interests?
Dr. Michael Hart’s (MH): My lab aims to define the role of genes and genetic variants in the generation of behaviors at the molecular, neuronal, and circuit levels.” Using a variety of genetic approaches in C. elegans, his lab studies the interactions between conserved neurodevelopmental and neuropsychiatric genes, as well as the environment. Ultimately, he hopes to “use C. elegans as a system for high throughput screening of genes, variants, and small molecules using behavior as a primary endpoint.
Dr. Eric Joyce (EJ): Our lab uses several model systems, including Drosophila, mouse, and human cells, to determine how chromosomes are functionally organized in 3-D space and time. We also develop and utilize new technologies that use fluorescent in situ hybridization (FISH) to interrogate chromosome organization at single-allele resolution.
Dr. Guo-Li Ming’s (GLM): Our research focuses on understanding the molecular mechanisms underlying neurodevelopment and how its dysregulation may contribute to developmental neurological disorders. Using two complementary model systems, the genetically modified mouse system and human induced pluripotent stem cell (hiPSC) derived 2D neural cells and 3D brain organoid model systems, we are interested in addressing a broad range of topics, from neuronal migration, axon and dendritic development, synapse formation, circuitry integration to plasticity of developing neurons, and functional regeneration of mature neurons.
Tell us about your model systems.
MH:Caenorhabditis elegans is a species of nearly transparent nematode that lives around the globe, eating bacteria in rotting vegetation. Sydney Brenner and colleagues pioneered C. elegans in the 1970’s as a model organism for research in neurobiology and development. C. elegans are transparent, primarily hermaphrodites that are ~1mm in length with 959 somatic cells and a ~3 day life cycle; all features that have allowed for pioneering and Nobel prize-awarded discoveries. This includes the discoveries in cellular organ development, programmed cell death, RNA interference, use of green fluorescent protein, and countless neuronal and behavioral discoveries.
I started working with C. elegans in 2012 as a postdoc in Oliver Hobert’s lab at Columbia University. As a Penn Neuroscience graduate student in Aaron Gitler’s lab, I learned the power of genetic model organisms through the use of yeast genetics and screening to study neurodegenerative diseases and protein aggregation. This experience led me to seek out a simple model organism that had neurons, which ended up being C. elegans!
GLM: Organoids are self-assembled three-dimensional cell aggregates generated from stem cells. In the lab, we are using hiPSCs derived from either healthy individuals or patients as the starting material to generate brain organoids. We have developed protocols to generate brain region specific organoids, including dorsal forebrain organoids, midbrain organoids, hypothalamus organoids, Arcuate Nucleus organoids, hippocampal organoids and choroid plexus organoids, applying these unique models to understand human brain development as well as neurological disorders.
EJ: The biggest advantage to using Drosophila as a model system is its rich history. Decades of reagent and tool building along with a thoughtful community of researchers have made Drosophila a super powerful gene discovery tool. As a result, many impactful screens in flies have been done over the years, revealing pathways governing essentially all fundamental cellular processes. If the gene has an odd or funny name, it was probably discovered in flies.
Why did you choose to work with these model systems?
MH: Of the 959 cells in C. elegans, 302 are neurons! C. elegans’ small nervous system is the most well-defined of any animal – with every neuron and synapse mapped in complete neuronal connectomes from hatching to adulthood. C. elegans generate many quantifiable behaviors that can be defined, in some cases, to the level of single genes functioning in single neurons. This resolution, together with C. elegans’ adaptability to amazing genetic and neuroscience tools, allows one to ask questions nearly impossible in other organisms. The affordability and fast life cycle make C. elegans ideal for high throughput forward and reverse genetics, allowing one to make fundamental discoveries for many genes in the generation of many behaviors, not to mention many other cellular and biological processes. A major limitation of C. elegans is that not all human genes and signaling pathways are conserved.
EJ: We mainly use Drosophila as part of our research program to identify novel genes implicated in 3D genome organization. Another huge advantage for us is that they only have 3 major chromosomes, allowing us to visualize with imaging tools the entire genome with relative ease. This is virtually impossible in mammalian systems.
GLM: There are considerable differences in the size, cellular diversity, and synaptic properties of the brains between humans and model systems. We and other labs have shown that human brain organoids recapitulate key characteristic features of human fetal nervous system development, including multiple human-specific features such as human-specific stem cells and astrocytes as well as diverse neuronal types.
Brain organoids have expanded our toolset for studying human brain development and mechanisms underlying many neurological diseases, but they remain imperfect model systems as there are several important caveats, including limited protocol reproducibility amongst different hPSC lines, heterogeneity between organoids, lack of definitive methods to accurately assess cell identity, and absence of structures that fully recapitulate the organization of the human brain.
How do you see use of this model system changing over time?
MH: While I have only been working with C. elegans for about 9 years, the biggest changes are in the expanding number of researchers using C. elegans and the precision with which researchers can ask questions, permitted by the development of incredible new genetic and neuroscience tools and technologies, including CRISPR/Cas9. These developments have strengthened research in C. elegans, where our detailed knowledge of the system allows implementation of the tools at high resolution (i.e. single neurons). The perception of C. elegans research seems similar to me over the last decade, with both skeptics and champions of C. elegans work across all research communities; I can’t count how many times I have been asked if C. elegans even have behavior. However, I think this is similar for most systems, including mice and other vertebrates. Where this may matter most is at funding agencies, where perceptions can create discrepancies in funding between different organisms.
EJ: As tools such as CRISPR make genetics research easier and faster in vertebrate systems compared to the past, one perception is that invertebrate systems aren’t as necessary. However, invertebrate systems such as Drosophila are still the best research bang for your buck for tax payer’s money. This plus the speed at which research can be conducted in a developmental system means that model system genetics isn’t going anywhere for a while. Instead, we are seeing a trend where labs are moving towards the use of multiple model systems, especially as research becomes more collaborative in general.
GLM: The perceptions regarding hiPSC-based 2D cellular and organoid models have changed for sure. I still remember the comments from one reviewer on our first paper using neurons derived from hiPSCs as a model to study mechanisms of schizophrenia. This reviewer basically did not believe that these derived neurons were neurons. Now, it is clear that more and more labs, including industry, are accepting organoids as models and platforms for basic biology, disease understanding, drug development and cell replacement therapy.
What is the translational value of your system?
MH: The biggest translational strength of C. elegans is in its ability to uncover fundamental conserved biology that translates in unexpected ways, whether the research was originally aimed at translation or not. While any given discovery in C. elegans may not have an immediate value to human health, they often snowball over years into techniques, molecular mechanisms, or conserved biology that impacts translational science and human health. For research directly aimed at translation, the adaptability and speed of C. elegans research means one can study many things in very high-throughput on an organism level, including genes, small molecules, genetic variants, etc.
Diversity of every kind strengthens the scientific enterprise, and this certainly extends to the use of model systems/organisms. Each model has unique questions that can be asked, or in the way they can be asked, and this should dictate our use of these organisms. Our goal should be to use each model system to answer scientific questions in complementary and orthogonal ways.
EJ: As I mentioned above, Drosophila is a powerful gene discovery tool that has uncovered and characterized many human disease genes and associated pathways. More recently, however, the simplicity of screening in Drosophila is proving to be a powerful mechanism to isolate drugs or drug targets for many human genetic disorders that can be appropriately modeled. I think this is an area that is going to see rapid growth in the next few years.
GLM: Currently, brain organoids have been used to study the potential clinically relevant phenotypes associated with various diseases and to identify potential druggable targets. As all model systems, there are limitations and advantages when talking about translational value. But I do have confidence in the translational value of brain organoids.
Why is your model system better than others?
MH: Did Michael Eisen (@mbeisen) put you up to this question? If so, I don’t understand the question and I won’t respond to it.
But really C. elegans is the best because of an exhaustive list of firsts – first metazoan cellular lineage, first neuronal connectome, first complete genome sequence, first olfactory ligand/receptor pair, and many more. Trainees that want to make a major ‘first’ discovery should choose C. elegans!
GLM: [Our organoids are] of human origin and we are superior?
Anything else you’d like use to know about your model system?
MH: Penn and CHOP have an amazing C. elegans research community that includes labs using C. elegans across many disciplines and using a wide variety of cool techniques – the include the labs of Meera Sundaram, John Murray, Colin Conine, David Raizen, Chris Fang-Yen, and Marni Falk
GLM: Further improvement of the brain organoid technology will allow us to understand the human brain in ways that were previously impossible, to facilitate precision medicine and to improve regenerative medicine.
Comments